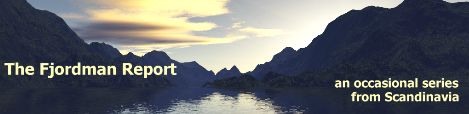
The noted blogger Fjordman is filing this report via Gates of Vienna.
For a complete Fjordman blogography, see The Fjordman Files. There is also a multi-index listing here.
This is Part 2 of a three-part series. Part 1 may be found at the Brussels Journal.

Since more than 70 percent of the Earth’s surface is covered by seawater, detailed studies of the oceans were of great importance to science as well as to practical navigation. The first modern text devoted exclusively to marine science was Histoire physique de la mer (1725) by the Italian military man and naturalist Luigi Ferdinando Marsigli (1658-1730), who assembled information about water temperature, salinity, currents, ocean plants and animals. The eighteenth century witnessed an acceleration of this trend as European explorers charted distant lands and the science of chemistry was rapidly maturing in Europe itself.
Ocean currents engaged the curiosity of scholars such as Benjamin Franklin who in the 1780s mapped the Gulf Stream, which originates in the Gulf of Mexico and brings vast amounts of warm water across the Atlantic Ocean to northwestern Europe, substantially contributing to the regional climate and the relatively mild winters there. Benjamin Thompson, Count Rumford’s heat experiments led him to attribute ocean circulation to differences in water density, a theory which was accepted after some delay. The English geographer James Rennell (1742-1830) served in the British Royal Navy, where his numerous voyages allowed him to make accurate maps and charts of currents and tides. His final work Currents of the Atlantic Ocean was a landmark study published posthumously by his daughter in 1832.
Matthew Fontaine Maury (1806-1873) of the United States Navy in 1842 became superintendent of the U.S. Depot of Charts and Instruments in Washington D.C. From the study of old ships’ captains logs Maury assembled data on winds, currents and other information and finally published his Physical Geography of the Sea in 1855, widely considered the first extensive textbook of oceanography. The Scots-Canadian oceanographer John Murray (1841-1914) was the first person to use the term “oceanography” and along with the Norwegian marine zoologist Johan Hjort (1869-1948) in 1912 published the influential book The Depths of the Ocean. The Norwegian oceanographer Harald Sverdrup (1888-1957) developed a comprehensive theory of ocean circulation.
By the early twentieth century, several persons had suggested the existence of some form of continental drift, but it was the German meteorologist and geologist Alfred Wegener (1880-1930) who had the biggest impact. He had studied at the universities of Heidelberg, Innsbruck and Berlin. From 1906 to 1908 he worked as a meteorologist to a Danish expedition to Greenland. In 1910 he had been struck, as others had been before him, of how Africa and South America seemed to “fit together.” It was known that surprisingly similar fossils and landforms could sometimes be found on opposite sides of major oceans. Based on these findings Wegener proposed his theory of continental drift in 1915 in his masterwork The Origin of Continents and Oceans (Die Entstehung der Kontinente und Ozeane). He suggested that there had once been a giant continent which he named “Pangaea” (“All-Earth”).
Scientists currently believe that Pangaea split up a little over 200 million years ago. These ideas are now widely accepted; in fact, it is believed that the major continents have drifted apart and been reunited in supercontinents several times with intervals of some hundreds of millions of years. However, it took generations for this theory to be accepted, in part because scientists lacked sufficient information about the ocean floors and in part because Wegener himself could not properly explain how continental drift happens or what drives it.
In the late nineteenth century, the invention of reasonably accurate and compact seismographs spurred the development of seismology into a quantitative discipline. In 1880 the Englishman John Milne (1850-1913), the inventor of the first modern seismograph, as a foreign advisor to the Meiji government founded the Seismological Society of Japan. Scientists in earthquake-ridden Japan soon joined the Germans and the Americans as world leaders in geophysics.
The Japanese geophysicist Motonori Matuyama (1884-1958) was the son of a Zen abbot. After studies at the Imperial University in Kyoto, he worked in Chicago with the American geologist Thomas Chamberlin (1843-1928). Matuyama proposed that long periods had existed in the geological past in which the polarity of the magnetic poles was the opposite of what it is now. A geomagnetic reversal is a change in the orientation of Earth’s magnetic field where magnetic north and south become interchanged. In the past 4 million years there have been nine reversals, which leave magnetic imprints in certain rock samples.
The English geologist Arthur Holmes was a proponent of Wegener’s continental drift. His pioneering work on radioactive heat and geological time had led him to a profound understanding of processes in our planet’s interior. He proposed that very slow-moving convection currents in the Earth’s mantle cause continental breakup, seafloor formation and continental drift. The Dutch geophysicist Felix Andries Vening Meinesz (1887-1966) measured gravity anomalies above the ocean floors. Before the 1940s, most geologists had assumed that the sea floor represented the most ancient crust. When samples where finally obtained from the ocean beds it turned out that they were far younger than expected and that the youngest samples were found next to the volcanically active mid-ocean ridges.
The existence of a “mountain range” in the middle of the Atlantic Ocean had been suspected since the laying of the first transatlantic telegraph cable in 1858, but the global system of mid-ocean ridges was mapped only after 1950. Both the United States and the Soviet Union, the latter with less financial resources at their disposal than the former, needed to known more about the ocean environment to navigate with their nuclear submarines. The seas constituted an important frontline in their Cold War superpower rivalry. Research coupled with electronic computers greatly increased the understanding of oceanography and atmospheric physics and facilitated the integration of these various fields into the umbrella discipline of Earth science.
Studies of paleomagnetism (changes in the Earth’s magnetic field “fossilized” in samples of magnetic minerals) and distribution of fossils triggered a mental revolution in geology and the emerging Earth sciences. The American geologist Harry Hammond Hess (1906-1969) while working as an officer in the US Navy during WWII conducted his own research of the ocean floors using the transport’s sounding gear. In 1960 at Princeton University, Hess put the pieces together and advanced the theory that the Earth’s crust moves laterally from volcanically active oceanic ridges. “Sea-floor spreading” helped to establish the concept of continental drift as scientifically respectable. The Canadian geophysicist John Tuzo Wilson (1908-1993) soon after created the new synthesis we know as plate tectonics.
According to scholars Philip Rehbock and Gary Weir, “Wegener proposed that the present configuration of the continents, and other phenomena from stratigraphy, paleontology, and biogeography, could be accounted for by assuming the gradual movement of the continents horizontally over the face of the globe. The theory gained few adherents until the 1960s, by which time new lines of evidence helped bring about the plate-tectonics revolution. Evidence came from studies of paleomagnetism and polar wandering carried out by P. M. S. Blackett, S. Keith Runcorn, and their colleagues in Britain; heat flow from mid-ocean ridges, by British geophysicist Edward Bullard; seismological activity along mid-ocean ridges, by Americans Maurice Ewing and Bruce Heezen; and gravity anomalies, by the Dutch geophysicist Felix Andries Vening-Meinesz and the American Harry H. Hess. In 1960, Hess proposed the hypothesis, subsequently known as sea-floor spreading, that would explain all of these phenomena. In the mid-1960s, the British geophysicists Frederick Vine and Drummond Matthews confirmed the hypothesis by analyzing patterns of magnetic anomalies around mid-ocean ridges, and the Glomar Challenger drilled directly into the Mid-Atlantic Ridge. J. Tuzo Wilson’s 1965 concept that the earth’s surface consists of several rigid but mobile plates put the finishing touch on plate tectonics.”
If two of these enormous plates are moving away from each other, an ocean ridge or continental ridge will form. If they collide, one may be pushed under the other in a process called subduction. If neither plate can subduct under the other they push up mountain ranges. The Mid-Atlantic Ridge, which is straddled by volcanically active Iceland, has churned out magma (fluid molten rock) and expanded the Atlantic for millions of years. The Eurasian plate collides with the African plate in the Mediterranean region, which is why the eastern Mediterranean in particular is a volcanically and seismologically active area with volcanoes Mount Etna on Sicily and Mount Vesuvius east of Naples. In the Aegean Sea, the massive Thera eruption at the island of Santorini around 1600 BC weakened the Minoan civilization.
Whereas the Alps in Central Europe were born through the collision between the African and European plates the Himalayas in Asia, the planet’s highest mountain range, were created by a collision between the Eurasian Plate and the Indo-Australian Plate. The so-called Pacific Ring of Fire is a region plagued by frequent earthquakes and volcanic eruptions. The western Pacific Ocean contains the deepest trench on the Earth’s crust, the Mariana Trench, which lies at the subduction boundary between two tectonic plates.
While most of the volcanic activity on our planet can be explained by plate tectonics there are nevertheless some exceptions. A hot spot is an area of persistent volcanic activity which originates at unusually hot areas of the mantle-core boundary in the Earth’s interior. Examples of such hot spots would be Galápagos, the unique Yellowstone area in the USA and Hawaii. The Hawaiian Islands are actually peaks of a great undersea mountain range created by the slow movement of a tectonic plate across a hotspot. Whereas the famous Mount Everest in the Himalayas stretches 8,848 meters above sea level, Mauna Kea in Hawaii would be over 10,000 meters if measured from the base of the mountain at the bottom of the ocean.
The theory of plate tectonics triggered a revolution in the Earth sciences in the late twentieth century. There are those who view it as a paradigm shift like the ones described by the American philosopher Thomas Kuhn (1922-1996) in his influential work The Structure of Scientific Revolutions from 1962. Kuhn broke with several key positivist doctrines held by philosophers of science such as Karl Popper and was responsible for popularizing the term “paradigm.” According to him, science enjoys periods of stable progress punctuated by revolutions when one conceptual world view is replaced by another. Kuhn received some criticism for these views by scholars who claimed that it is more appropriate to describe the development of social and cultural ideas than the history of science; some postmodern thinkers have used or misused his ideas to claim that there is no such thing as objective truth.
- - - - - - - - -
While the theory of plate tectonics is now almost universally accepted we still don’t know how this process started. Scientists believe that it began at least 2.5 billion, possibly as much as 4 billion years ago. The very young Earth was probably too hot for the crust to solidify completely and the lightest minerals would have floated to the surface. Geologist Vicki Hansen of the University of Minnesota in a controversial hypothesis from 2007 suggested that the impact of a massive asteroid or comet might have kick-started plate tectonics on Earth.
Stones falling from the sky were often viewed by ancient peoples as signs from the gods. In Enlightenment Europe, stories about such events were usually dismissed by scholars as common superstition. The German scholar Ernst Chladni (1756-1827), who is often regarded as the founder of meteoritics, in 1794 published a paper suggesting the extraterrestrial origin of meteorites, asserting that masses of iron and rock enter the atmosphere from above and produce fireballs when heated by friction with the air. He concluded that they must be cosmic objects. This view was defended by the German astronomer Heinrich Olbers (1758-1840), but ridiculed by those who believed meteorites were of volcanic origin and created on Earth.
Eyewitness accounts of fireballs were initially dismissed, yet fresh and seemingly reliable reports of stones falling from the sky appeared at the turn of the nineteenth century. The young English chemist Charles Howard (1774-1816) read Chladni’s work and decided to analyze the chemical composition of these rocks. Working with the French mineralogist Jacques-Louis de Bournon he made the first thorough scientific analysis of meteorites. Here is a quote from the book Cosmic Horizons, edited by Neil De Grasse Tyson and Steven Soter:
“The two scientists found that the stones had a dark shiny crust and contained tiny ‘globules’ (now called chondrules) unlike anything seen in terrestrial rocks. All the iron masses contained several percent nickel, as did the grains of iron in the fallen stones. Nothing like this had ever been found in iron from the Earth. Here was compelling evidence that the irons and rocks were of extraterrestrial origin. Howard published these results in 1802. Meanwhile, the first asteroid, Ceres, was discovered in 1801, and many more followed. The existence of these enormous rocks in the solar system suggested a plausible source for the meteorites. Space wasn’t empty after all. Finally, in 1803, villagers in Normandy witnessed a fireball followed by thunderous reverberations and a spectacular shower of several thousand stones. The French government sent the young physicist Jean-Baptist Biot to investigate. Based on extensive interviews with witnesses, Biot established the trajectory of the fireball. He also mapped the area where the stones had landed: it was an ellipse measuring 10 by 4 kilometers, with the long axis parallel to the fireball’s trajectory. Biot’s report persuaded most scientists that rocks from the sky were both real and extraterrestrial.”
The French physicist Jean-Baptiste Biot (1774-1862) also did work on the polarization of light and contributed to electromagnetic theory. He accompanied the great chemist Joseph Gay-Lussac in 1804 on the first balloon flight undertaken for scientific purposes, reaching a height of several thousand meters while doing research on magnetism and the atmosphere. The Montgolfier brothers had performed the first recorded manned balloon flight in France in 1783. The French meteorologist Léon Teisserenc de Bort (1855-1913) later discovered the stratosphere, the layer of the Earth’s atmosphere above the troposphere which contains most of the clouds and weather systems, by using unmanned, instrumented hydrogen balloons. The French physicist Charles Fabry (1867-1945) discovered the ozone layer in the upper atmosphere in 1913. Ozone (O3) is tri-atomic oxygen that exists in the stratosphere as a gas and protects us from most of the harmful effects of ultraviolet radiation from the Sun.
Unmanned gas balloons are still used for meteorological, scientific and even military purposes. Without a pressurized cabin, manned ballooning becomes dangerous in the upper reaches of the atmosphere due to the cold, the low pressure and particularly the lack of oxygen. The Swiss inventor Auguste Piccard (1884-1962), who served as a professor of physics in Brussels, created balloons equipped with pressurized cabins and set a number of flight records during the 1930s, reaching an altitude of 23,000 meters.
His son Jacques Piccard (1922-2008), a Brussels-born Swiss oceanographer, explored the deepest reaches of the oceans when he and the American Don Walsh (born 1931) in 1960 used the bathyscaphe Trieste to travel 10,900 metres down to the bottom of the Challenger Deep in the Mariana Trench in the western Pacific. The pressure exerted by ten meters depth of water roughly equals one atmosphere. At the bottom of the Challenger Deep the pressure is consequently well over one thousand times the standard atmospheric pressure at sea level, yet amazingly Piccard and Walsh spotted fishes living even under these extreme conditions.
The French naval officer, explorer, ecologist, author and prizewinning filmmaker Jacques-Yves Cousteau (1910-1997) invented the aqualung together with the engineer Émile Gagnan (1900-1979) in 1943. Cousteau was a pioneer in the development of underwater cameras as well. He possessed the rare gift of being able to communicate his love of the natural world to a mainstream audience and did a great deal to popularize knowledge of underwater biology.
The Austrian physicist Victor Francis Hess (1883-1964), educated at the Universities of Graz and Vienna, in a series of balloon ascents in 1911-13 established that radiation increases with altitude. Early explorers of radioactivity studied its intensity from church steeples and tall buildings. Hess found that radiation declined during the first 1,000 m of ascent but then began to rise again, reaching double that at surface level at 5,000 m. By flying his balloon at night and during a solar eclipse he demonstrated that this radiation comes from outer space but not from the Sun. This high-energy ionizing radiation is now called cosmic rays.
The troposphere contains about 80% of the total mass of the atmosphere with most of the rest concentrated in the next layer, the stratosphere. The air temperature in the troposphere drops with altitude. The depth of this layer varies from about 8 to 16 kilometers from the polar regions to the warm tropics. Commercial airliners typically cruise at altitudes of above 10 km to optimize jet engine fuel burn and stay above most of the turbulent weather found below.
From an altitude of 11 to 50 kilometers above the Earth’s surface we find the stratosphere. The lower portion of this layer is influenced by the polar jet stream and subtropical jet stream, fast uniform winds concentrated in a narrow band. The stratosphere defines a layer in which temperatures rises with increasing altitude. This is caused by the absorption of ultraviolet (UV) radiation from the Sun by the ozone layer. Weather balloons filled with lighter-than-air gases such as hydrogen or helium may reach the stratosphere but cannot explore the layers above it as the decreasing pressure causes the balloons to expand until they disintegrate.
The mesosphere stretches from 50 km to 80 km. Temperatures here drop with increasing altitude to almost -100°C, making this the coldest atmospheric layer. The ionosphere can be found at an altitude of around 80 km, at the border to the thermosphere. Solar radiation here generates very high temperatures but the air is extremely thin. There is no specific height at which the atmosphere begins or ends; it gradually merges into space. Nevertheless, the Kármán line, named after the Hungarian American physicist Theodore von Kármán (1881-1963), at 100 km above sea level is sometimes used to mark the beginning of space. The International Space Station (ISS), a research laboratory in a microgravity environment and probably the most expensive man-made object ever created, is currently found in a Low Earth Orbit roughly 340 kilometers above the Earth’s surface. Artificial satellites are usually not put into orbit at altitudes of less than 300 km as this is considered impractical.
Space is full or rocks, ranging from asteroids to specimen the size of a fist. A meteoroid is too small to be called an asteroid or a comet. Even smaller particles are called cosmic dust grains. If meteoroids enter our atmosphere they become meteors. Most meteors burn up by the friction before they reach the ground, typically in the mesosphere where they create fireballs we see as shooting stars. Those who survive this fiery entry become known as meteorites. Many meteorite specimens are believed to be remnants of the Solar System as it was during its formation, most of them probably fragments of asteroids. Based on their chemical composition, a few known meteorites are believed to come from the Moon or the planet Mars.
The French geochemist and mining engineer Gabriel Daubrée (1814-1896) developed a classification system for meteorites and their composition. In 1866 he presented his theory that the Earth has a nickel-iron core, similar to the nickel-iron alloys we can find in certain iron meteorites. In early, hot planetary bodies iron-rich metal and other heavy elements may have separated from the molten bodies to form dense cores inside shells of silicate material.
As we have seen, the nebular hypothesis was presented in the eighteenth century by Emanuel Swedenborg, Immanuel Kant and Pierre-Simon Laplace. The planets and asteroids all revolve around the Sun in the same direction and roughly the same plane, which strongly indicates that they were created during the same process. In 1905 the geologist Thomas Chamberlin and astronomer Forest Ray Moulton (1872-1952) in the USA developed a theory that smaller objects, planetesimals, orbited the Sun during the formation of the Solar System and collided to build the larger planets. Some elements of their theory remain in use today.
We currently believe that about 4.6 billion years ago the solar nebula, a cloud of interstellar dust and gas, was slowly spinning in a flat rotating disk. Many objects in the Oort cloud and the Kuiper belt may be rocky chunks known as planetesimals left over from this period. Small chunks of matter collided to form kilometer-sized planetesimals, some of which collided further to form a few hundred planetary embryos and moons. It is possible that one of these protoplanets slammed into the very young Earth and thereby created our Moon; the Solar System certainly was a much more turbulent place back then than it is today. Well over 99 % of the material in the solar nebula gathered to form a protostar, until the temperature and pressure at the core was high enough to start hydrogen fusion and give birth to our Sun.
The Italian astronomer Giuseppe Piazzi (1746-1826) studied at Milan, Turin and Rome, taught philosophy for a time at Genoa and mathematics at the University of Malta. Well-connected in Italy and within the European scientific community he managed to establish a decent observatory at Palermo, Sicily. In 1801 Piazzi discovered Ceres, the first known asteroid. He correctly believed it to lie in the orbital region between Mars and Jupiter but soon fell ill and lost track of the object. Based on the very limited number of observations the brilliant young German mathematician Carl Friedrich Gauss successfully calculated its orbit.
Ceres is by far the most massive body in the asteroid belt. Its size is sufficient to give it a spherical shape and it is therefore considered a “dwarf planet.” It contains a significant quantity of water ice and probably preserves a record of what the Solar System was like when it was first condensing from cosmic dust into planetesimals and larger protoplanets. Many astronomers believe that it was the gravitational influence of the neighboring gas giant Jupiter that prevented the plantesimals of the asteroid belt for form into a regular planet.
Soon after Piazzi’s discovery the German physician and astronomer Heinrich Olbers found two more asteroids, Pallas and Vesta. Next to his medical practice in Bremen, Olbers conducted astronomical observations and discovered several comets. He is also famous for popularizing Olbers’ Paradox, a question which scholars had found surprisingly difficult to answer: Why is the sky dark at night? If the universe is eternal and contains an infinite number of stars then presumably it should be much lighter than it is. The preferred answer to this, at least within the framework of the current Big Bang cosmological model, is that the universe is not infinitely old (less than 14 billion years old) and that it is expanding. Partial credit for providing the correct answer to this riddle has been given to the American author Edgar Allan Poe (1809-1849) as well as to the great British physicist Lord Kelvin.
After the introduction of photography, the German astronomer and astrophotographer Max Wolf (1863-1932) from the University of Heidelberg invented a technique for revealing asteroids by the streaks they left on photographic plates, thus discovering hundreds of new ones. Astronomers then discovered the asteroid belt between the planets Mars and Jupiter and by extension found an explanation for many impact craters on various planetary bodies.
The discipline of Earth science was invented in the 1960s and 70s when it replaced geology as the major discipline for studying our planet, just as geology had once replaced mineralogy. Geophysicists, geologists, oceanographers and meteorologists began working on related problems using similar techniques and implements. At the same time the first space probes were being sent to investigate other bodies in our Solar System, which meant that geologists could extend the scope of their investigations to the domain formerly dominated by astronomers. This led to the creation of planetary science and branches such as astrobiology.
The American geologist and astronomer Eugene Shoemaker (1928-1997) was one of the pioneers of planetary science and arguably founded astrogeology. In much of his asteroid and comet work he collaborated with his wife Carolyn Shoemaker (born 1929), who currently holds the record for most comet discoveries by any one individual with 32. They were co-discoverers of Comet Shoemaker-Levy 9, whose spectacular collision with Jupiter in 1994 was followed closely by scientists. Eugene Shoemaker did much to bring attention to the significance of possible impacts from comets and asteroids during the Earth’s history.
We know from the fossil record that there have been several mass extinctions of life on our planet previously, but the causes of them remain disputed. Many people believe that the extinction which ended the age of the dinosaurs around 65 million years ago was at least partly caused by the impact of a large asteroid or comet. The American experimental physicist and Nobel laureate Luis W. Alvarez (1911-1988) from the University of California, Berkeley together with his son Walter Alvarez (born 1940) suggested this theory in 1980.
One of the key pieces of evidence was a clay layer (the K-T boundary) from this period which contains an unusually high concentration of the platinum metal iridium. Iridium is very rare in the Earth’s crust because it is dense and therefore presumably sank into the core during the Earth’s childhood. It is much more abundant in meteorites and by extension probably in asteroids and comets. Since then, a huge impact crater from this geological period has been identified outside of the Yucatán Peninsula in Mexico, although doubts have been raised by some scholars as to whether this impact was the sole cause of the mass extinction in question.
No comments:
Post a Comment
All comments are subject to pre-approval by blog admins.
Gates of Vienna's rules about comments require that they be civil, temperate, on-topic, and show decorum. For more information, click here.
Users are asked to limit each comment to about 500 words. If you need to say more, leave a link to your own blog.
Also: long or off-topic comments may be posted on news feed threads.
To add a link in a comment, use this format:
<a href="http://mywebsite.com">My Title</a>
Please do not paste long URLs!
Note: Only a member of this blog may post a comment.